Exploring Jupiter's Outer Magnetosphere
This project will focus on the outer regions of Jupiter's magnetosphere, where spacecraft observations often reveal a transition between the periodically oscillating plasma sheet / magnetodisc of Jupiter, and a region known as the 'cushion' which is comparatively devoid of a continuous plasma sheet, but for which observational evidence suggests it is a region where individual blobs of plasma are detaching from the outer edge of the plasma disc.
On the modelling side, the project will focus on improving outer magnetodisc structure of our in-house UCL Magnetodisc model, by looking at different methods for 'shielding' the magnetic field inside a model of the magnetospheric boundary.
The improved model will then be used as a tool to look at outer magnetospheric passes from spacecraft such as Galileo (mission completed and data archived) and Juno (still active), in order to answer questions such as:
- where, how and how often does the cushion region appear?
- what does the modelling of the data tell us about the corresponding distribution of plasma and magnetospheric currents, and the role of the 'bracing' of the field by the magnetopause boundary?
The project thus represents an opportunity to learn more about interpretation of magnetometer and plasma data from spacecraft, and the use of mathematical models, based on MHD equilibria, in interpreting such data.
Contact: Prof Nick Achilleos (nicholas.achilleos AT ucl.ac.uk)
The Planetary X-rays Revolution
For decades, the X-ray waveband has been significantly underutilised in the study of planetary systems, yet it provides vast untouched scientific opportunity. X-rays offer unique and essential insights into the composition of planetary atmospheres and moons (important for habitability and formation), planetary aurora and magnetic fields (critical for protecting planets from the stellar winds of their partner star), and the energetic environment surrounding planets.
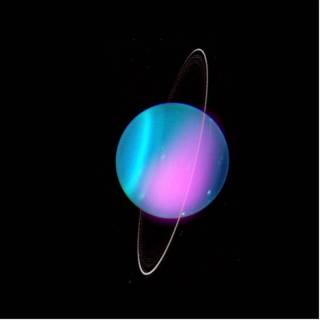
Overlaid Chandra X-ray (purple) and Keck IR observation of Uranus. X-ray: NASA/CXC/UCL/W.Dunn et al, IR: Keck
UCL are the world-leaders in the X-ray study of the outer planets systems. To address the shortages of planetary X-ray studies, the NASA and ESA flagship observatories (Chandra and XMM-Newton) have awarded our group the largest dataset of planetary X-ray observations ever acquired. This includes: the first X-ray observation of an interstellar comet; 1000+ hours of X-ray observations of Jupiter and its moons, coordinated with the Juno spacecraft (including the first ever spatially resolved Jupiter XUV observation) and 120 hours of X-ray observations of Uranus planned contemporaneous with JWST and Hubble (dwarfing our previous few-hour observation that was reported in the international media last year). Most of this data is untouched and rich with potential for new and transformative discoveries and our group has a strong track record for publishing in Nature and Science journals.
The observations above were acquired with Earth-orbit X-ray observatories. However, recent developments in X-ray instrumentation mean that lightweight X-ray instruments can now be flown to the planets. This planetary X-ray revolution begins in 2025 with the ESA-JAXA BepiColombo mission to Mercury and the ESA-CAS SMILE mission for Earth observation. We are currently working with partners at NASA, ESA and CAS to define and lead the first X-ray instrumentation to the outer planets and their moons. We also led the planetary working group for the new ~$bn X-ray probe, the Line Emission Mapper, which is currently in phase 1 consideration by NASA. If selected, we will be ideally positioned to obtain the first planetary observations with the telescope.
Students will have the opportunity to take key roles in developing the science for future NASA/ESA/CAS mission concepts and proposals (currently: LEM; COMPASS and Tianwen-4 spacecraft proposals). In conjunction with this, we have very strong collaborations in the US, Europe and Asia, so that students will work as part of a global space science community.
Contact: Dr William Dunn (w.dunn AT ucl.ac.uk)
Refining interpretability of machine learning models for exoplanet characterization via improved uncertainty estimation
Machine learning (ML) algorithms are driving innovation across domains, exoplanetary science among them. Two important yet often overlooked aspects are model interpretability and uncertainty quantification. Most popular ML algorithms (e.g. deep neural networks, ensembles), are notorious for being 'black boxes', but several model interpretability methods (of various degrees of reliability) have been developed to allow us to understand their inner workings, to uncover hidden biases in the models (or the data), to increase trustworthiness and adoption, to inspect when and how they fail, or to uncover new domain knowledge. Similarly, popular ML algorithms are also known to suffer from poor uncertainty estimation -crucial for cost-sensitive applications and for prioritizing objects for further analysis. Regression models often fail to provide cohesive confidence intervals and classification models often ignore class membership probabilities -or obtain systematically unreliable estimates thereof. Techniques to address these issues include conformal prediction and probabilistic calibration.
In this project, we will investigate if these two weaknesses are connected and by addressing one, we improve upon the other. ‘Can we make machine learning models more interpretable by improving how they quantify uncertainty over their predictions?’ We will explore this and related questions in the context of ML models for inferring atmospheric parameters from spectra obtained from extrasolar planets (exoplanet characterization).
Contact: Dr. Nikolaos Nikolaou (n.nikolaou AT ucl.ac.uk)
NEXOS: Non-LTE spectroscopy of exoplanetary atmospheres
Exoplanet research is a rapidly expanding field that offers a fascinating glimpse into the diversity of celestial bodies in our universe. The study of exoplanetary atmospheres is particularly intriguing as it sheds light on complex properties such as 3D effects, climates, weather, and chemical non-equilibrium, including non-local thermodynamic equilibrium (non-LTE) effects. While much of the research on exoplanet atmospheres assumes local thermodynamic equilibrium (LTE), non-LTE effects have been detected in Earth's atmosphere, the atmospheres of other solar system planets, stars, comets, and the interstellar medium. The non-LTE effects can be crucial in the middle and upper atmospheres of (exo-)planets and influence strongly the absorption and especially the emission emerging from these layers. However, despite the importance of non-LTE effects, their modelling remains a challenging field of research and currently the interpretation of the exoplanetary atmospheric observations do not account for deviations from LTE.
The STFC funded project NEXOS aims to initiate and advance the non-LTE studies of exoplanetary atmospheres by developing a comprehensive solution for characterisation and detection of non-LTE effects in atmospheric spectra of exoplanets. Specifically, NEXOS will focus on integration of non-LTE modeling of molecular spectroscopy into mainstream exoplanetary retrieval codes as a self-consistent solution of the statistical equilibrium and radiative transfer equations. NEXOS will fill the critical gaps in the provision of the molecular opacities required for adequate non-LTE solutions, for the key molecules of the modern exoplanetary atmospheric applications using advanced molecular quantum mechanical methods. Through forward modelling of atmospheric spectra of exoplanets, it will test non-LTE scenarios for known exoplanets and those that will be discovered in the future and identify most promising manifestations of non-LTE both in ground based and space exoplanetary observations.
Contact: Prof. Sergey Yurchenko (s.yurchenko AT ucl.ac.uk)
Spectroscopy of Exoplanets
Thousands of exoplanets have been discovered in the recent years. These newly-discovered planets are generally unlike those in our Solar System. Some of the rocky super-Earths are evaporating with complex atmospheric compositions. These planets have a lot in common with the young Earth; the massive amounts of water in their atmospheres can melt rocks and put their constituents into the atmosphere. Similar processes are expected in the atmospheres of the post-impact planets. A prerequisite for advances to be made is the availability of the fundamental atomic and molecular data necessary for interpreting new observations. The unusual conditions found on most known exoplanets, involving elevated temperatures and high fluxes of stellar radiation, means the required data are missing and not readily measurable in the laboratory.
This PhD project will aim to produce comprehensive molecular opacities specific to lava-planets using advanced molecular quantum mechanics allied to high-performance computing in response to the modern challenges of exoplanetary models and retrievals. The results will be incorporated into exoplanet models developed at UCL and made available to the scientific community via the ExoMol database. These models will enable the interpretation of present and future spectroscopic studies of rocky super-Earths. Exactly these types of hot solid planets will be the likely targets of NASA's JWST or ESA's Ariel.
Contact: Prof. Sergey Yurchenko (s.yurchenko AT ucl.ac.uk)
ExoMolHD
The ExoMol team at UCL is the world leader in providing spectroscopic data for the characterisation and modelling of exoplanets and other hot atmospheres, see http://www.exomol.com. The ERC-funded ExoMolHD project is dedicated to providing high quality spectroscopic data. We have an open position to work on one of the following tasks:
1. Generation of molecular line lists for astronomical studies;
2. Providing precise wavelengths for key molecules applicable for use in high resolution spectroscopic studies performed by telescopes using high resolution instruments;
3. Predicting accurate spectroscopic data on key isotopically-substituted species;
4. Providing temperature-dependent pressure shifts and pressure broadening parameters;
5. Computing photodissociation cross sections and photolysis rates both in and outside thermodynamic equilibrium;
6. Developing appropriate database structures, including detailed opacities, k-tables and precomputed atmospheric models.
We will act to ensure the widest possible utilisation of the data.
Contact: Prof. Sergey Yurchenko (s.yurchenko AT ucl.ac.uk) and Prof. Jonathan Tennyson (j.tennyson AT ucl.ac.uk)
Pandemonium in the planetary graveyard
Defying the notion of the silent graveyard, planetary systems refuse to go quietly into the long night. Instead, a significant fraction show one or more signs of dynamical reanimation, with strong indications of general mayhem during the final stages of stellar evolution. These rejuvenated planetary systems manifest as irregular and complex transit events, transient optical emission features, and variable infrared fluxes from dust production and destruction. Ultimately, all leave their detailed chemical signatures on the surface of the white dwarf stars they orbit, and provide powerful insight into the masses and geochemical structures of the planetary bodies. At UCL, we are leading the study of these evolved planetary systems in the infrared via their dusty debris disks, in the optical via transiting events, and in the ultraviolet where elemental abundances can be measured from the polluted stellar surfaces. The project will involve at least two observational approaches, including but not limited to: studies of available transit data, infrared data that track debris disk variability, and importantly bulk compositions for minor and major planetary bodies.
Contact: Prof Jay Farihi (j.farihi AT ucl.ac.uk)
Unveiling the nature of super-Earths with current and future observatories
Super-Earths, i.e. planets lighter than ten Earth masses, appear to be the most common planets in our galaxy. Being absent in our Solar Systems, their nature is rather mysterious: from their densities we gather there is a large variety of cases, ranging from big rocky planets to small Neptunes or more exotic types. The chemical composition and state of their atmospheres, can be used as a powerful diagnostic of the history, formation mechanisms and evolution of these planets. In the past fifteen years, the UCL exoplanet team led by Prof. Tinetti has worked at the forefront of the spectral/photometric measurements of exoplanet atmospheres and their interpretation, with molecular species being detected in the atmospheres of giant planets and super-Earths (e.g. extremely hot 55 Cnc-e and habitable-zone K2-18b). As part of the PhD, the student will have the opportunity to work in collaboration with Prof. Giovanna Tinetti, Dr. Angelos Tsiaras and Dr. Yuichi Ito on a number of aspects connected with the observations and modelling of super-Earths’ atmospheres with current and future observatories (HST, JWST) and dedicated space missions (ARIEL).
Contact: Prof Giovanna Tinetti (g.tinetti AT ucl.ac.uk, also cc e.dunford AT ucl.ac.uk)
Planetary chemical composition and its origins in protoplanetary disks
In the 2020s, exoplanet studies are increasingly focussing on planetary chemical composition and its origins in protoplanetary disks. Some of the key goals are to link planetary elemental abundances to their formation location and migration history in a disk, and to establish the origins of habitable chemical compositions. Of particular interest are the astro- and biochemically important elements carbon, hydrogen, nitrogen, oxygen, phosphorus, and sulfur (CHNOPS). Questions also abound concerning the potential chemical diversity of Earth-like worlds, or the origins and nature of various types of planets such as hot Jupiters or super-Earths. To tackle the above problems, we must study the composition, structure, and processes in planet-forming environments.
In my group, we mainly focus on studying protoplanetary disks, using our own observations and models (e.g. Kama et al. 2016; Keyte et al. 2022; Chen et al. 2023), but also contributing in large, international ALMA programmes such as DECO which provide a wealth of data on protoplanetary disk composition. We are also a partner in the EXOHOST project, which studies disk- and planet-hosting stars (see e.g. Jermyn & Kama 2018; Kama et al. 2019, 2023), and involved in the upcoming Ariel mission. Ariel is led from UCL and will launch in 2029 to characterise the composition of ~1000 planets.
PhD projects are available in my group to work on planet formation science using revolutionary instruments such as the ALMA interferometer or JWST; on the physical-chemical modelling of planet formation processes and environments; and on the connections between planetary systems, their natal disks, and their host stars.
Contact: Dr Mihkel Kama (m.kama AT ucl.ac.uk)`